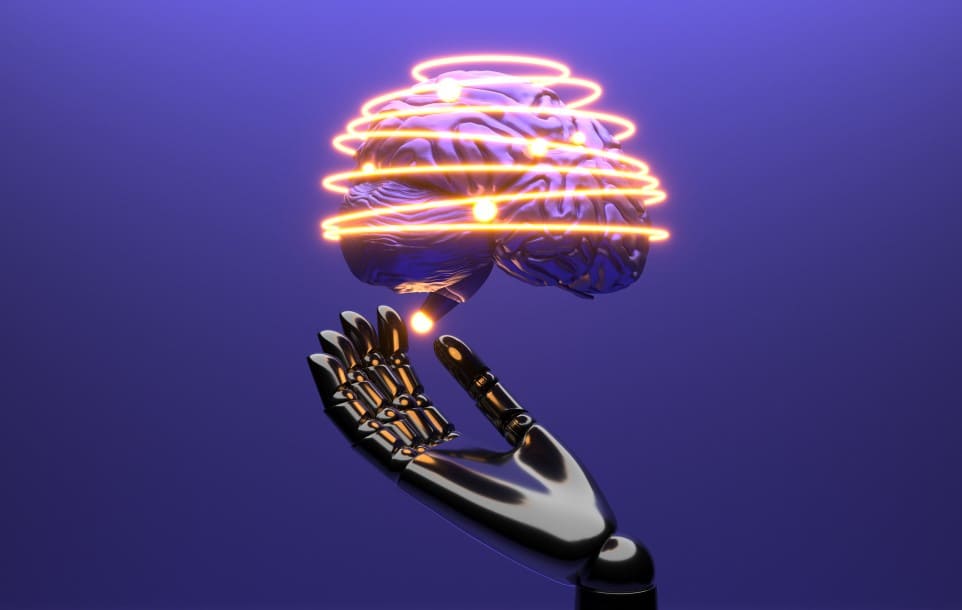
Overview:
To understand what is a Brain-Computer Interface (BCI), we need to explore a revolutionary advancement at the intersection of neuroscience and technology. By creating direct communication pathways between the brain and external devices, BCIs offer unprecedented possibilities for enhancing human capabilities and interactions. This cutting-edge technology has the potential to transform various sectors, from healthcare and communication to gaming and smart environments. By translating neural signals into commands, BCIs can empower individuals with disabilities, enhance cognitive functions, and provide new ways to interact with the digital world.
The journey of BCIs began in the mid-20th century with early explorations into brain signal recording and interpretation. Over the decades, significant technological and scientific advancements have propelled BCIs from experimental concepts to practical applications. Today, BCIs are at the forefront of innovation, driving forward the possibilities of human-machine integration. This article explores the evolution of BCIs, their key applications across different sectors, the benefits they offer, the challenges they face, and the exciting future they promise to shape.
Contents:
- What is a Brain-Computer Interface (BCI)
- Understand Brain-Computer Interface (BCI) in layman’s term
- Evolution of Brain-Computer Interfaces (BCIs) with notable milestones and developments
- How a Brain-Computer Interface (BCI) works
- Key application areas of Brain-Computer Interfaces (BCIs)
- Key benefits of Brain-Computer Interfaces (BCIs)
- Key challenges of Brain-Computer Interfaces (BCIs)
- The futuristic outlook on Brain-Computer Interfaces (BCIs)
- Summing Up
What is a Brain-Computer Interface (BCI):
A Brain-Computer Interface (BCI) is a technology that enables direct communication between the brain and an external device. BCIs can be used to restore lost sensory or motor functions, enhance existing capabilities, or provide new interactions between humans and machines.
Let’s understand what a Brain-Computer Interface (BCI) is in layman’s term:
A Brain-Computer Interface (BCI) is a technology that allows a person to control a computer or other devices just by using their brain. Think of it as a special connection between your brain and a machine. This connection lets you communicate with the machine without needing to move your body. It’s like using your thoughts to tell a computer what to do.
For example, someone could use a BCI to play a video game, control a wheelchair, or even type on a screen, all by thinking about what they want to happen. This technology is especially useful for people who can’t move or speak easily, giving them a new way to interact with the world.
The evolution of Brain-Computer Interfaces (BCIs) with notable milestones and developments over the years:
1960s – Early Conceptualization:
- Researchers begin exploring the idea of direct communication between the brain and machines.
- Hans Berger’s discovery of the electroencephalogram (EEG) in the 1920s lays the groundwork for future BCI research by demonstrating that brain waves can be recorded from the scalp.
1970s – Initial Experiments:
- Jacques Vidal coins the term “Brain-Computer Interface” in a 1973 paper and conducts experiments demonstrating the feasibility of controlling a cursor on a screen using EEG signals.
- Early studies focus on understanding and mapping brain signals and their potential applications.
1980s – Development of Basic Systems:
- Advances in computing and signal processing allow for more sophisticated BCIs.
- Researchers begin to develop systems that can interpret basic commands from brain signals to control simple devices.
1990s – Proof-of-Concept and Applications:
- Researchers at the University of California, Los Angeles (UCLA), and other institutions develop more advanced BCIs capable of more complex tasks.
- Philip Kennedy implants the first human BCI in a paralyzed patient, allowing them to control a computer cursor.
2000s – Commercial and Medical Applications:
- The first commercial BCI systems are developed, primarily for medical applications such as assistive technologies for individuals with disabilities.
- Companies like Cyberkinetics develop the BrainGate system, which allows paralyzed individuals to control computers and robotic arms using their thoughts.
- Researchers achieve significant milestones in non-invasive BCIs using EEG for applications in gaming and communication.
2010s – Expansion and Diversification:
- BCIs see broader applications beyond medical uses, including gaming, virtual reality, and smart home control.
- Advances in machine learning and artificial intelligence improve the accuracy and functionality of BCI systems.
- Notable projects like DARPA’s “Revolutionizing Prosthetics” program push the boundaries of BCI technology, developing advanced prosthetic limbs controlled by thought.
- Companies like Neuralink, founded by Elon Musk in 2016, aim to develop high-bandwidth brain-machine interfaces for a range of applications, from medical treatments to human enhancement.
2020s – Integration and Innovation:
- BCIs become more integrated with other technologies, such as artificial intelligence, robotics, and the Internet of Things (IoT).
- Continued advancements in non-invasive BCI technologies make them more accessible and practical for everyday use.
- Neuralink demonstrates significant progress in developing implantable BCI devices, with the goal of achieving human trials.
- Research focuses on improving the usability, reliability, and safety of BCIs, making them viable for a broader range of users and applications.
Now, let’s look at how a Brain-Computer Interface (BCI) works:
Usually it is a five-step process
1. Signal Acquisition:
1.A Non-Invasive Methods:
Electroencephalography (EEG): EEG uses electrodes placed on the scalp to measure electrical activity produced by brain neurons. It is commonly used due to its non-invasive nature and relatively low cost.
Functional Near-Infrared Spectroscopy (fNIRS): fNIRS measures changes in blood flow in the brain using near-infrared light. It is also non-invasive and can provide information about brain activity.
1.B Invasive Methods:
Electrocorticography (ECoG): ECoG involves placing electrodes directly on the surface of the brain. This method provides high-quality signals but requires surgical implantation.
Microelectrode Arrays: These arrays are implanted in the brain tissue and can record signals from individual neurons. They offer the highest precision but involve significant surgical risks.
2. Signal Processing:
Preprocessing: The raw neural signals are often contaminated with noise from muscle movements, electrical interference, and other sources. Preprocessing steps like filtering, artifact removal, and normalization are applied to clean the data.
Feature Detection: Algorithms are used to detect relevant features in the neural signals. For example, specific frequency bands in EEG signals, such as alpha (8-12 Hz) or beta (13-30 Hz) waves, might be analyzed for their correlation with certain mental states.
3. Feature Extraction:
Time-Domain Features: Characteristics like the amplitude and duration of the neural signals are examined over time.
Frequency-Domain Features: The power spectrum of the signals, which shows the distribution of power across different frequency bands, is analyzed.
Spatial Features: The spatial distribution of neural activity across different regions of the brain is considered.
4. Translation Algorithm:
Machine Learning Models: Various machine learning techniques, such as support vector machines (SVMs), neural networks, and deep learning models, are employed to learn the relationship between neural features and intended actions.
Calibration: The system is often calibrated for each user, requiring them to perform specific tasks while the BCI learns to recognize their neural patterns. For example, a user might repeatedly imagine moving their left or right hand to train the system.
5. Device Output:
The translated commands can be used to control a variety of external devices. For instance:
Computer Cursors: Users can move a cursor on a screen by imagining specific movements, like moving their hands.
Prosthetic Limbs: BCIs can control robotic arms or legs, allowing users to perform tasks such as grasping objects or walking.
Communication Devices: For individuals with severe disabilities, BCIs can enable communication by selecting letters on a virtual keyboard or generating synthetic speech.
Smart Home Systems: Users can control lights, TVs, and other smart home devices using neural commands, enhancing accessibility for people with limited mobility.
Key application areas of Brain-Computer Interfaces (BCIs):
1. Healthcare and Medicine
1.A Neuroprosthetics:
Applications: Prosthetic limbs, cochlear implants, retinal implants.
Details: BCIs allow individuals with amputations or paralysis to control artificial limbs by translating neural signals into movements. Cochlear implants use BCIs to convert sound into electrical signals that stimulate the auditory nerve, helping those with hearing loss. Retinal implants aim to restore vision by stimulating the optic nerve.
1.B Communication Devices:
Applications: Speech-generating devices, computer interfaces.
Details: BCIs enable individuals with severe speech and motor impairments to communicate by translating brain signals into text or speech through specialized software, allowing them to interact with others and access information.
1.C Neurorehabilitation:
Applications: Robotic exoskeletons, virtual reality environments.
Details: BCIs are used in rehabilitation to help stroke or spinal cord injury patients regain motor function. Robotic exoskeletons can be controlled by brain signals to assist in walking and movement therapy. Virtual reality environments provide immersive rehabilitation exercises controlled by thought.
1.D Neurofeedback Therapy:
Applications: ADHD, anxiety, depression treatments.
Details: Neurofeedback involves training individuals to regulate their brain activity. BCIs monitor brainwaves and provide real-time feedback, helping users learn to control their mental state and improve symptoms of ADHD, anxiety, and depression.
1.E Mood Monitoring and Regulation:
Applications: Mood tracking devices, therapeutic interventions.
Details: BCIs can monitor emotional states by analyzing brainwave patterns. This data can be used to develop personalized therapeutic interventions and mood regulation techniques, aiding in the treatment of mood disorders and enhancing overall well-being.
2. Gaming and Entertainment
2.A Virtual Reality (VR) and Augmented Reality (AR):
Applications: Interactive VR/AR systems, immersive experiences.
Details: BCIs enhance VR and AR experiences by allowing users to interact with virtual environments through thought commands. This creates more natural and engaging interfaces, improving the level of immersion and interaction in gaming and entertainment.
2.B Neurogaming:
Applications: Brain-controlled games, adaptive gaming environments.
Details: Neurogaming leverages BCIs to create games that respond to the player’s brain activity. This allows for personalized gaming experiences that can adapt in real-time to the player’s mental state, providing a unique and engaging way to play.
3. Education and Training
3.A Cognitive Training:
Applications: Memory improvement programs, attention enhancement tools.
Details: BCIs can be used to develop cognitive training programs that adapt to the user’s brain activity, helping improve memory, attention, and other cognitive skills. These programs can be personalized based on real-time feedback from the BCI.
3.B Skill Acquisition:
Applications: Learning aids, performance monitoring tools.
Details: BCIs monitor brain activity during skill learning and provide feedback to optimize the learning process. This can accelerate the acquisition of new skills, such as playing a musical instrument or mastering a new language, by tailoring the training to the individual’s cognitive state.
4. Research and Development
4.A Neuroscience Research:
Applications: Brain mapping, functional connectivity studies.
Details: BCIs are valuable tools in neuroscience research, allowing scientists to study brain function and connectivity in detail. This research can lead to a better understanding of neural mechanisms and the development of treatments for brain disorders.
4.B Human-Computer Interaction (HCI):
Applications: Advanced user interfaces, adaptive computing systems.
Details: BCIs contribute to the development of advanced HCI technologies, aiming for more intuitive and efficient interactions between humans and machines. This includes adaptive computing systems that respond to the user’s cognitive load and emotional state.
5. Security and Authentication
Biometric Authentication:
Applications: Secure access systems, personalized security solutions.
Details: BCIs can be used for biometric authentication by analyzing unique brainwave patterns. These patterns serve as a secure identifier, providing access to systems and devices only to authorized users.
6. Smart Environments and Internet of Things (IoT)
6.A Home Automation:
Applications: Smart home control systems, environmental adjustments.
Details: BCIs enable individuals to control smart home devices through thought commands. This includes managing lighting, temperature, and other aspects of the home environment, enhancing convenience and accessibility.
6.B Wearable Technology:
Applications: Health monitoring devices, fitness trackers.
Details: BCIs integrated with wearable technology can provide continuous monitoring of personal health and fitness parameters. This data can be used to track physical activity, monitor vital signs, and provide insights into overall health.
7. Mental Health and Well-being
7.A Neurofeedback:
Applications: Self-regulation training, mental health therapy.
Details: Neurofeedback with BCIs helps individuals learn to control their brain activity, which can be beneficial for treating mental health conditions. By providing real-time feedback, users can develop skills to self-regulate their mental state.
7.B Mood Monitoring and Regulation:
Applications: Personalized therapeutic interventions, well-being enhancement tools.
Details: BCIs can track and help regulate emotional states, offering personalized therapeutic interventions for mood disorders. This can include strategies to enhance well-being and manage stress more effectively.
Key benefits of Brain-Computer Interfaces (BCIs):
1. Improved Quality of Life
Restoration of Lost Functions: BCIs can help individuals with disabilities regain lost sensory or motor functions, significantly enhancing their independence and quality of life.
Enhanced Communication: For individuals with severe communication disorders, BCIs provide a means to interact with others and the environment, reducing isolation and improving social interactions.
2. Medical Advancements
Advanced Prosthetics: BCIs enable more natural and intuitive control of prosthetic limbs, improving the functionality and acceptance of these devices.
Neurorehabilitation: Patients recovering from strokes or spinal cord injuries can benefit from BCI-driven therapies that promote neuroplasticity and functional recovery.
3. Mental Health and Cognitive Benefits
Neurofeedback and Therapy: BCIs provide real-time feedback on brain activity, helping individuals learn to regulate their mental states, which can be beneficial for managing conditions like ADHD, anxiety, and depression.
Cognitive Enhancement: BCIs can be used to develop training programs that enhance cognitive functions such as memory, attention, and learning capabilities.
4. Innovations in Human-Computer Interaction
Hands-Free Control: BCIs allow users to control computers and other devices without the need for physical input, which is particularly useful for individuals with severe physical disabilities.
Adaptive Systems: BCIs enable the development of adaptive user interfaces that respond to the user’s cognitive load and emotional state, making interactions more efficient and intuitive.
5. Enhanced Gaming and Entertainment Experiences
Immersive Gaming: BCIs create more immersive and interactive gaming experiences by allowing players to control games with their thoughts.
Personalized Content: Games and entertainment systems can adapt in real-time to the user’s mental state, providing a more engaging and personalized experience.
6. Security and Authentication
Biometric Authentication: BCIs can provide a highly secure method of authentication based on unique brainwave patterns, reducing the risk of unauthorized access.
7. Smart Environment Control
Home Automation: BCIs can control smart home devices, enhancing convenience and accessibility for individuals with mobility issues.
IoT Integration: BCIs can be integrated with IoT devices for seamless control and monitoring of various aspects of personal and home environments.
8. Scientific Research and Knowledge Expansion
Brain Function Insights: BCIs offer valuable tools for neuroscience research, helping to uncover the mechanisms of brain function and connectivity.
New Therapeutic Approaches: Research with BCIs can lead to the development of new treatments for neurological and psychological disorders.
9. Increased Accessibility
Empowering Disabled Individuals: BCIs provide new opportunities for people with disabilities to participate in daily activities, work, and social interactions, promoting inclusivity and equality.
Assistive Technologies: BCIs can enhance the capabilities of existing assistive technologies, making them more effective and easier to use.
10. Personalized Health Monitoring
Continuous Monitoring: BCIs integrated with wearable technology can provide continuous monitoring of health parameters, offering early detection of potential issues and personalized health insights.
Proactive Healthcare: Real-time data from BCIs can support proactive healthcare interventions, improving outcomes and reducing healthcare costs.
Key challenges of Brain-Computer Interfaces (BCIs):
1. Technical Challenges
Signal Acquisition: Obtaining high-quality, reliable neural signals is difficult due to noise and interference. The brain’s electrical signals are weak and easily affected by external factors, requiring advanced technologies for accurate capture.
Data Processing: Interpreting complex brain signals accurately and quickly is challenging. Sophisticated algorithms and significant computational power are needed to process and decode these signals in real-time.
2. Invasiveness and Safety
Invasive Procedures: Some BCIs require surgical implantation, which poses risks such as infection, inflammation, and other surgical complications. Non-invasive methods are generally safer but often less accurate.
Long-Term Effects: The long-term impact of using BCIs, particularly invasive ones, is not fully understood. Continuous monitoring and research are necessary to ensure they are safe for prolonged use.
3. User Training and Adaptation
Learning Curve: Users often need extensive training to effectively use BCIs. Adapting to controlling devices with brain signals can be time-consuming and mentally demanding.
User Variability: Individual differences in brain structure and function can affect BCI performance. A system that works well for one person may not work as effectively for another, necessitating personalized calibration.
4. Ethical and Privacy Concerns
Data Privacy: BCIs involve sensitive neural data, raising significant privacy issues. Ensuring that this data is protected from misuse and unauthorized access is crucial.
Ethical Dilemmas: The ability to read and potentially influence brain activity raises ethical concerns about consent, autonomy, and the potential for misuse or abuse of the technology.
5. Cost and Accessibility
High Costs: Developing and deploying BCI systems is expensive, involving advanced technology and specialized expertise. This can limit accessibility for many potential users.
Economic Disparities: High costs may exacerbate existing disparities in access to healthcare and technology, with advanced BCI solutions being available only to those who can afford them.
6. Integration and Compatibility
System Integration: Integrating BCIs with existing technologies and ensuring compatibility with various devices and platforms is complex. Seamless integration is necessary for widespread adoption.
Standardization: Lack of standardization in BCI technology and protocols can hinder interoperability and the development of universal solutions.
7. Regulatory and Approval Processes
Regulatory Hurdles: BCIs must undergo rigorous testing and obtain regulatory approval, which can be lengthy and complex. Ensuring that these devices meet safety and efficacy standards is crucial for gaining public trust.
Ethical Approval: Research involving BCIs, particularly invasive ones, requires ethical approval, which can be a time-consuming process due to the sensitive nature of the studies.
8. Public Acceptance and Perception
Skepticism and Fear: Public skepticism and fear about the potential risks and ethical implications of BCIs can hinder acceptance and adoption. Education and transparent communication are needed to address these concerns.
Stigma: There may be a stigma associated with using BCIs, particularly for those who require them for medical reasons, which can affect users’ willingness to adopt the technology.
9. Performance and Reliability
Accuracy: Ensuring high accuracy in interpreting neural signals is challenging, especially for complex tasks. Misinterpretation can lead to errors in control and functionality.
Consistency: Maintaining consistent performance over time and across different conditions is difficult, as neural signals can vary due to factors like fatigue, stress, and environmental influences.
The futuristic outlook on Brain-Computer Interfaces (BCIs):
1. Seamless Human-Machine Integration
Symbiotic Relationships with AI: BCIs could enable a seamless integration between human cognition and artificial intelligence, allowing for real-time information exchange, decision-making, and enhanced cognitive abilities. Humans might collaborate with AI on complex tasks, leveraging the strengths of both biological and artificial intelligence.
Enhanced Reality Perception: BCIs could directly interface with the brain’s sensory pathways, creating highly immersive augmented and virtual reality experiences that feel indistinguishable from real life. This could revolutionize entertainment, education, and remote work.
2. Cognitive and Physical Enhancement
Superhuman Capabilities: BCIs might enhance human abilities beyond natural limits, such as improved memory, faster learning, heightened sensory perception, and even telepathic communication between individuals.
Mind-Controlled Exoskeletons: Advanced BCIs could enable mind-controlled exoskeletons or robotic suits, giving humans superhuman strength and endurance. This could be particularly useful in fields such as construction, disaster response, and space exploration.
3. Medical Revolution
Cure for Neurological Disorders: BCIs could potentially cure or significantly alleviate neurological disorders such as Alzheimer’s, Parkinson’s, and epilepsy by directly interfacing with and modulating neural circuits.
Regenerative Medicine: Integration with stem cell technology and tissue engineering could allow BCIs to facilitate the repair and regeneration of damaged neural tissues, leading to groundbreaking treatments for spinal cord injuries and neurodegenerative diseases.
4. Communication and Social Interaction
Telepathic Communication: BCIs might enable direct brain-to-brain communication, allowing people to share thoughts, emotions, and sensory experiences without the need for language. This could revolutionize interpersonal communication and understanding.
Global Brain Network: A future “neural internet” could emerge, where individuals can connect their minds to a global network, sharing knowledge and experiences on an unprecedented scale, leading to a collective intelligence far surpassing current capabilities.
5. Space Exploration
Enhanced Astronaut Capabilities: BCIs could enhance the capabilities of astronauts, allowing them to control spacecraft and robotic systems with their minds, adapt to the stresses of space travel more effectively, and communicate telepathically across vast distances.
Remote Colonization: Mind-controlled robotic systems and avatars could enable humans to explore and colonize distant planets without physically being there, using BCIs to control these systems from Earth or a nearby spacecraft.
6. Immortality and Consciousness Transfer
Mind Uploading: Advances in BCI technology might one day make it possible to upload and transfer human consciousness to digital platforms or other biological hosts, potentially achieving a form of digital immortality.
Brain-Cloud Interface: A direct interface between the human brain and cloud computing resources could allow individuals to store and access their memories, skills, and knowledge externally, enhancing cognitive functions and preserving personal identity beyond biological constraints.
7. Ethical and Societal Transformations
Redefining Humanity: As BCIs become capable of enhancing and altering fundamental aspects of human experience, society will need to address profound ethical questions about identity, agency, and the nature of being human.
Equity and Access: Ensuring equitable access to BCI technology will be crucial to prevent socio-economic divides and ensure that the benefits of these advancements are shared broadly across society.
8. Environmental and Ecological Impact
Enhanced Environmental Monitoring: BCIs integrated with sensor networks could enable humans to monitor and respond to environmental changes in real-time, leading to better conservation efforts and disaster response.
Sustainable Living: Mind-controlled systems could optimize resource usage in smart cities, homes, and agricultural systems, promoting more sustainable living practices and reducing the human ecological footprint.
Summing Up:
Brain-Computer Interfaces (BCIs) have progressed remarkably since their early conceptual stages, transforming from basic experimental setups to sophisticated systems with wide-ranging applications in healthcare, communication, and entertainment. These interfaces have provided unprecedented opportunities for individuals with disabilities, enabling them to regain lost functions and communicate more effectively. BCIs have also enhanced human-computer interaction, offering new levels of control and immersion in virtual environments. Despite these advancements, challenges such as technical complexities, ethical concerns, and high costs remain significant barriers to widespread adoption and accessibility.
Looking forward, the future of BCIs is filled with transformative potential. Integration with artificial intelligence could lead to enhanced cognitive abilities, direct brain-to-brain communication, and even possibilities like mind uploading. Such advancements could fundamentally alter human capabilities and societal structures, blurring the lines between biology and technology. To realize these benefits, it is crucial to address the existing challenges thoughtfully and ensure equitable access. By fostering responsible development and considering ethical implications, BCIs can pave the way for a new era of human enhancement and technological integration, promising a future where the synergy between human minds and machines creates profound new possibilities.